Difference between revisions of "テザードメンブレン"
(→膜タンパク) |
(→膜タンパク) |
||
Line 43: | Line 43: | ||
グラミジン [http://en.wikipedia.org/wiki/Gramicidin gramicidin] (1882 Da) やバリノマイシン [http://en.wikipedia.org/wiki/Valinomycin valinomycin] (1111 Da),などの最も小さいイオンチャネルであるポリペプチドなどは、テザードメンブレンの中に均一に配位されます。 | グラミジン [http://en.wikipedia.org/wiki/Gramicidin gramicidin] (1882 Da) やバリノマイシン [http://en.wikipedia.org/wiki/Valinomycin valinomycin] (1111 Da),などの最も小さいイオンチャネルであるポリペプチドなどは、テザードメンブレンの中に均一に配位されます。 | ||
− | 図 3 に示すような大きなイオンチャネルでは、上部('superstructures' | + | 図 3 に示すような大きなイオンチャネルでは、上部('superstructures')がメンブレン近傍の水溶液の中まで伸びています。中位のサイズのタンパクでさえ、その 'superstructures' は tethaPlasm の中に納まることは稀で、(生きた細胞で見られるように)膜タンパクの好ましい配位を取ります。 |
== Ion Channels == | == Ion Channels == |
Revision as of 18:51, 27 May 2014
Contents
テザードメンブレンとは?
テーザードメンブレンは金電極の表面に リン脂質二重層 をサポートする平坦膜で、一対の疎水性 ポリエチレングリコール (PEG) 鎖がアンカーと呼ばれる有機ジスルフィド によって金表面に共有結合しています(図 1 参照)。親油性のアルカン フィタル基がPEG鎖の上部で結合し、それが骨格として働き周辺の脂質膜をクラスター化させ、強いては連続膜を形成するまでになります。
PEG鎖のジスルフィドアンカーと共に、フィタル基 も膜を金表面に結合させるのでテザー 分子と呼ばれます。実際には、金基質上でテザー同士はスペーサと呼ばれる親油性の フィタニル基の無い類似の分子を介して離れています。これらのスペーサは膜の下にあり、直接膜には接していません。金基質表面はテザーとスペーサとで被覆されますが、 両者がくっつくことは無く、大きな有機ジスルフィドアンカー基を挟んで離れています。
テザーとスペースとの平均間隔は約4 nm (およそ水分子9個分)で、金表面上の膜の厚さは約40 nm (但し、0.5 nmは有機ジスルフィドアンカが占めています)です。
従って膜と金表面間のスペースはPEG鎖、ジスルフィドアンカ群、様々なカチオンやアニオン、及び水分子の混合体で、これを総じてtethaPlasm™と呼んでいます。 これは生きた細胞のサイトプラズマ (細胞質)に相当し、サイトプラズマのようにバルク水とは若干異なる特性を示します。
一定のバイアス電圧を用いて剰余カチオンやアニオンを有するこの tehaPlasm を'チャージ'して、tethaPatch や tehaPod の測定に利用します (下記参照)。
テザードメンブレンはタフです!
テザーとスペーサとの混合比は製造過程で異なります。標準のtethaPlate™ は膜の安定性や柔軟性が最も高いとされる1:10の割合で提供されています。 高い柔軟性が求められる場合は 1:100 程度の割合まで使えますが、これ以下では膜の品質が損なわれ推奨できません。逆に100%まで上げると、安定性が最大限得られます。
混合比 10% の標準のテザードンブレンは極めて頑丈で、調整後数ケ月のライフタイムを持っています(4°C で保存すること)。膜は電気パルスに対しても極めて強く -500 ~ +800 mV の範囲の電位にも強い耐性を示しますので、従来のホールセルクランプの測定に用いられる以上の電圧をかけることも可能です。
tethaPlate™
tethaPlate™ は金電極 (3.0 x 0.7 mm = 2.1 mm2) を含む6つの各チャンバーから成り、金電極表面はテザーとスペーサ分子とで被覆処理されています。適切なリン脂質ミクスチャーを加えると自発的に膜を形成します。ついで未処理の金カウンター電極を膜の上 0.15 mm にセットしますが、膜には接触させません。
tethaPlate は極めて頑丈なハウジングなので、通常のイオンチャネル標本が数日程度しか保存できないのに比べて、シンプルなリン脂質で調整したテザード膜なら 4℃で数週間は安定して保存できます!
tethaPlate の取り付け方とテザードメンブレンの調整法は Video 1 と 2 をご覧ください。
膜タンパク
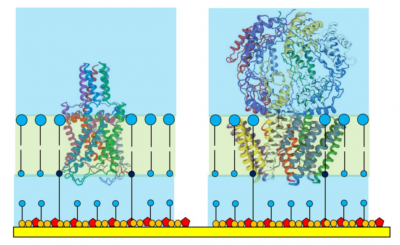
多くのタンパクは親油性部位と親水性部位から構成されており、こらがタンパクの三次構造(tertiary protein structure)に関与しています。 親油性部位はかなりの頻度で細胞や細胞小器官、膜と結合していますので、これらのタンパクは膜タンパク(membrane proteins) と呼ばれます。
ある種の膜タンパクは、比較的透過性のある親油性の細胞膜を通るカチオンやアニオンのトランスポートを促進します。このようなタンパクを イオンチャネル と呼びます。.
テザードメンブレンのテザー/スペーサの混合比で取り込めるタンパクの大きさが決まります。標準的な1:10の混合比では40,000 Da (言い換えれば、膜内の実際のタンパク部分)までのタンパクを取り込むのに適しているとされ、個々のタンパクはオリゴマーのポア構造に組み込まれ、最終的に一部は膜内でイオンチャンネルを形成します。
グラミジン gramicidin (1882 Da) やバリノマイシン valinomycin (1111 Da),などの最も小さいイオンチャネルであるポリペプチドなどは、テザードメンブレンの中に均一に配位されます。
図 3 に示すような大きなイオンチャネルでは、上部('superstructures')がメンブレン近傍の水溶液の中まで伸びています。中位のサイズのタンパクでさえ、その 'superstructures' は tethaPlasm の中に納まることは稀で、(生きた細胞で見られるように)膜タンパクの好ましい配位を取ります。
Ion Channels
Low molecular weight polypeptide ionophores and ion channels (such as gramicidin, valinomycin, magainin, alamethicin, and ionomycin) are available commercially in pure form. Larger proteins may be commercially available supplied in a detergent or lipid matrix. However most larger proteins must be custom made using bacterial culture, using genomic techniques either in-house or by a contract biotechnology company.
Typically the culture bacteria have the appropriate gene or RNA fragment artificially inserted into them so that they over express the desired ion channel protein. After several days of incubation the bacteria are harvested and the protein components separated and purified (often by gel electrophoresis). Whereas this was cutting edge technology in the 1990's these techniques are now well developed and widely available in proteomics laboratories.
The human genome itself is believed to code for about 400 different types of ion channel, and only a fraction of these have as yet been studied in detail. However, only a handful of ions - sodium potassium, calcium, magnesium, hydrogen, chloride (and other anions) - are relevant to the study of ion channels. The large variety of ion channels is required for different types of activity in different tissues. For example different potassium ion channels can be involved in action potential propagation in neve cells, the regulation of cardiac rythm, the response of cells to pressure changes (mechano-sensitive ion channels).
Similar ion channels occur in different organisms, however the structure is often slightly altered to optimise function as required by the species concerned.
Ionophores
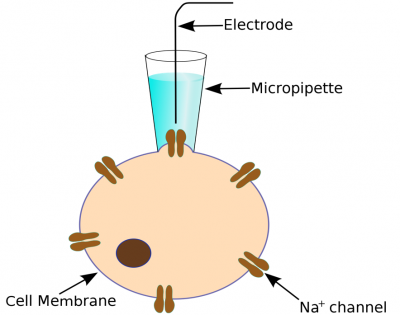
Both natural (such as ionomycin and calicimycin), and artificial ionophores (such as crown ethers, calixeranes, cryptands and other synthetic ion channels) can also be studied with tethered membrane systems. Ionophores are not always polypeptides and may be quite small molecules, however they must be at least partially lipohilic to ensure they can enter the membrane. Several ionophores have found applications as antibiotics, livestock growth promoters, and ion sensors.
Classic Patch Clamping
The classic patch clamp experiment uses a micropipette electrode that is positioned against a cell membrane to cover just one, or a few, ion channels. Usually a prolonged trial and error process is required to get the electrode in the right position. The ion current signal is normally very small (a few picoamperes) and great care must be take to achieve good signal/noise levels.
Variants of the technique include whole cell and oocyte patch clamping where the signals from many ion channels can be recorded simultaneously. This gives a larger (and quieter) signal but may be complicated by there being several types of ion channel present.
In recent years automated patch clamps have entered the market but the technique is still hampered by the difficulty of positioning a micropipette electrode to get reproducible signals.
Genomics/Proteomics Revolution
As mentioned above, the first years of the 21st century saw the raphid development of genomics and proteomics techniques so that it is now possible to isolate quantities of a single type of ion channel protein. This technology is now widespread in universities, research institutes, pharmaceutical company labs and hospital research departments.
For the first time studies can now be done on a 'routine' basis with reasonable quantities of pure protein giving a single type of ion channel. No longer are researchers restricted to making patches where only one or a several ion channel pores are being monitored.
The tethaPatch™
By assembling a single type of ion channel protein in a large patch (2.1 mm2 area) of artificial tethered membrane membrane somewhere between 1 and 10 million channels can be studied in parallel. The measured ion current is the sum total from all the ion channels in the patch, so that signals in the microampere ranges are obtained with concomitantly low noise levels. Because the ion channels spontaneously house themselves in the tethered membrane which is already bonded to the working electrode there is no 'hit-or-miss' approach with a micropipette electrode.
tethaPatch™ techniques, while analogous to whole cell patch clamping, typically provide results in minutes that would otherwise take hours with conventional techniques. Also because the tethered membranes are very robust, voltage pulses can be applied that would otherwise 'blow' the seal of a traditional patch clamp preparation. Thus voltage-gated, and mechanosensitive ion channels, can now be studied over a wide range of pulse amplitudes (potentials between -500 to +800 mV can be applied) than previously achievable (typically less than ±300 mV), leading to new insights into the mechanism of ion channel behaviour.
The ER466 Integrated Potentiostat is suitable for use with the tethaPatch, Figure 6, for pulse generation and data acquisition of the current signal.
The tethaPod™
tethaPod techniques take advantage of the relatively large signals provided by a tethered membrane system. By applying a small amplitude (20 mV) AC signal of varying frequency to the electrodes of a tethaPlate™, the membrane conductivity and capacitance can be directly determined. This is particularly relevant for pharmaceutical studies where continuous monitoring of membrane conductance can be done while various concentrations of ion channel blocker or actuator are added to the tethaPlate™. The results can then be analysed by dose/response methods enabling rapid screening of potential drug candidates.
References
- Comparative Study of the Bacterial Sodium Channel NaChBac Function using Patch Clamp and A.C. Impedance Spectroscopy in a Tethered Membrane. Bruce A. Cornell, Gwenael Scolan, Andrew M. Powl, Sonia Carne, Bonnie A. Wallace, Biophysical Journal, 102, 3, 338a, 2012. DOI: 10.1016/j.bpj.2011.11.1852
- The protective effect of osmoprotectant TMAO on bacterial mechanosensitive channels of small conductance MscS/MscK under high hydrostatic pressure. Evgeny Petrov, Paul R. Rohde, Bruce Cornell, and Boris Martinac. Channels, 6:4, 1-10, 2012. DOI: 10.4161/chan.20833
- Ion Channel Proteins that Spontaneously Insert into Lipid Bilayer Membranes: An Impedance Spectroscopy Study Employing Tethered Membranes. Bruce A. Cornell, Heba Alkhamici, Louise Brown, Sonia Carne, Sophia C. Goodchild, Russell Richards, and Stella M. Valenzuela. Biophysical Journal, 102, 682a-683a, 2012. DOI: 10.1016/j.bpj.2011.11.3710
- A High-Throughput Technique for Screening Novel Antibacterial Agents Targeting Bacterial Mechanosensitive Channels. Bruce A. Cornell, Andrew R. Battle, Sonia Carne, and Boris Martinac. Biophysical Journal, 102,122a, DOI: 10.1016/j.bpj.2011.11.683
- Making lipid membranes even tougher. Jognandan Prashar, Phillip Sharp, Mathew Scarffe, and Bruce Cornell. Journal of Materials Research, 22, 2189-2194, 2007. DOI: 10.1557/jmr.2007.0288
- Tethered Bilayer Membranes Containing Ionic Reservoirs: Selectivity and Conductance. Gowri Krishna, Jurgen Schulte, Bruce A. Cornell, Ron J. Pace, and Peter D. Osman. Langmuir, 19, 2294–2305, 2003. DOI: 10.1021/la026238d
- Tethered Bilayer Membranes Containing Ionic Reservoirs: The Interfacial Capacitance. Gowri Krishna, Jurgen Schulte, Bruce A. Cornell, Ron Pace, Lech Wieczorek, and Peter D. Osman. Langmuir, 17, 4858–4866, 2001. DOI: 10.1021/la001480a
- Tethered-bilayer lipid membranes as a support for membrane-active peptides. B. A. Cornell, G. Krishna, P. D. Osman, R. D. Pace and L. Wieczorek. Biochemical Society Transactions, 29, 613–617, 2001. DOI: 10.1042/bst0290613
- Tethered Lipid Bilayer Membranes: Formation and Ionic Reservoir Characterization. Burkhard Raguse, Vijoleta Braach-Maksvytis, Bruce A. Cornell, Lionel G. King, Peter D. J. Osman, Ron J. Pace, and Lech Wieczorek. Langmuir, 1998, 14, 648–659 1998. DOI: 10.1021/la9711239
Trademarks: tethaPod, tethaPatch, tethaPlate, and tethaPlasm are trademarks of SDx Tethered Membranes Pty Ltd.