EA164 QuadStat
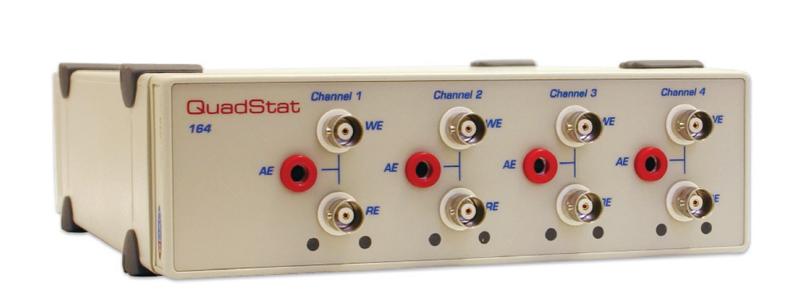
EA164 QuadStat
- Four-channel potentiostat 10mA to 200pA range
- Separate/common reference and auxiliary electrodes
- BiPotentiostat
- Ideal for amperometric biosensors, microbial fuel cells, photovoltaic materials and neurochemistry
The QuadStat is a software-controlled four-channel potentiostat with many Research Advantages (see below). Each channel can be used as a single three-electrode potentiostat. Alternatively two (bipotentiostat), three, or four working electrodes can be used in a single reaction chamber with a common reference and auxiliary electrode. Potential at each working electrode can be independently adjusted between ±2.5 V, or by using an external waveform generator to between ±10 V. The QuadStat can measure currents from pico- to milliamperes, making it ideal for amperometric biosensor research including use as a multichannel monitor for in vivo nitric oxide or dissolved oxgen sensors.
Two QuadStats can be 'piggybacked' to provide eight potentiostat channels.
By connecting the corresponding auxiliary and reference electrode leads, the QuadStat can be used as a four channel two-electrode (working and counter electrode) potentiostat. There is also a zero resistance ammeter (ZRA) mode of operation on each channel.
The QuadStat provides up to eight signals (four current and four potential signals) and is recommended for use with e-corder 821 or e-corder 1621 units. The QuadStat is normally sold as part of the ER7005 QuadStat Bundle.
Multichannel operation with included Chart software allows for:
- Simultaneous monitoring of sensors in multiple reaction vessels
- Bipotentiostat operation: two working electrodes with common auxiliary and reference electrode; also 3 or 4 working electrodes with common auxiliary and reference.
- Sensors: use with amperometric sensors providing current signals down to the picoampere ranges
- Neurochemistry: in vivo amperometry for neurotransmitter monitoring
Research Advantages
- Using Chart software you may record signals for very long time periods. The Quadstat system stores the data on the computer hard disk as it goes, so even in the event of a power outage data is saved.
- The recorded signals can be calibrated using one-, two-, or even multi-point calibration (up to twelve points) using linear or nonlinear correction. Removing the need to calibrate after obtaining the recording.
- As analyte additions are made (or other events occur) the use can enter notes directly into the recording and thus the notes will be tied to the signal at the relevant time. These notes are stored within the data file and can be easily recalled and displayed and searched as required.
- As a signal is being collected it is sometimes useful to review that signal and the Chart software supports a 'split window' so you can compare the incoming signal with a signal that was recorded at an earlier time. This allows for easy, on the fly, comparisons of previously recorded and actively recording data.
- The user may resize the signal on the computer screen while recording.
-
Users can start and stop recording, and change:
- gain sensitivity
- recording speed
- number of sensors being recorded
and keep all the new recordings in the one compacted data file. No proliferation of tens, or even hundreds, of separate disk files that have to be archived. All the recordings made on one day, or even a week, may be kept in a single data file that can be searched based on included notes. - If your signal comprises 'peaks' and you need to calculate and tabulate the peak heights and/or areas then you can use several Chart functions to perform this operation. Other features allow you to collect mean signal values (and standard errors) over defined time periods, evalute signal drift, or to fit the signal to idealised exponential or other functions, etc.All recorded signals may be copied and pasted as raw data into Excel (which Chart can also do) which may be needed to perform calculations. Just prepare the final table of results in Chart and copy them straight into a report.
Research Areas
Application Notes
- Cleaning and Polishing Voltammetric Electrodes
- Cyclic Voltammetry: Hints and Tips
- How to Choose a Potentiostat
Citations
- Electropolymerized phenol derivatives as permselective polymers for biosensor applications. Giammario Calia, Patrizia Monti, Salvatore Marceddu, Maria A. Dettori, Davide Fabbri, Samir Jaoua, Robert D. O’Neill, Pier A. Serra, Giovanna Delogu, and Quirico Migheli, Analyst, 140, 3607-3615, 2015. DOI: 10.1039/c5an00363f
- Quantitation of Cu+-catalyzed decomposition of S-nitrosoglutathione using Saville and electrochemical detection: a pronounced effect of glutathione and copper concentrations. Abdulghani Ismail, Sophie Griveau, Fanny d'Orlyé, Anne Varenne, and Fethi Bedioui. Electroanalysis, in press 2015. doi: 10.1002/elan.201500371
-
Nanostructured Platform Based on Graphene-Polypyrrole Composite for Immunosensor Fabrication. Andreea Cernat, Mihaela Tertiș, Claudia Nicoleta Păpară, Ede Bodoki, Robert Săndulescu, International Journal of Electrochemical Science, 10, 4718-4731, 2015. electrochemsci.org/papers/vol10/100604718.pdf
-
Marine sediments microbes capable of electrode oxidation as a surrogate for lithotrophic insoluble substrate metabolism. Annette R. Rowe, Prithiviraj Chellamuthu, Bonita Lam, Akihiro Okamoto and Kenneth H. Nealson, Frontiers in Microbiology, 5:784, 2015. doi: 10.3389/fmicb.2014.00784
-
Enriching distinctive microbial communities from marine sediments via an electrochemical-sulfide-oxidizing process on carbon electrodes. Shiue-Lin Li and Kenneth H. Nealson, Frontiers in Microbiology, 6:111, 2015. doi:10.3389/fmicb.2015.00111
-
A mediated turnip tissue paper-based amperometric hydrogen peroxide biosensor. Nadia Chandra Sekar, Liya Ge, Seyed Ali Mousavi Shaegh, Sum Huan Ng, Swee Ngin Tan, Sensors and Actuators B: Chemical, 210, 2015. doi: 10.1016/j.snb.2014.12.045
-
Carbon thin films as electrode material in neural sensing. Emilia Kaivosoja, Sami Sainio, Jussi Lyytinen, Tommi Palomäki, Tomi Laurila, Sung I. Kim, Jeon G. Han, Jari Koskinen, Surface & Coatings Technology, in press, 2014. doi: 10.1016/j.surfcoat.2014.07.056
-
Simple thick-film thread-based voltammetric sensors. Nadia Chandra Sekar, Seyed Ali Mousavi Shaegh, Sum Huan Ng, , Liya Ge, Swee Ngin Tan, Electrochemistry Communications, 46, 128–131, 2014. doi:10.1016/j.elecom.2014.07.003
-
Selective Detection of Dopamine against Ascorbic Acid Interference Using 3D Carbon Interdigitated Electrode Arrays. Rahul Kamatha and Marc J Madoub, ECS Transactions, 61, 65–73, 2014. doi: 10.1149/06107.0065ecst
-
Diamond-like carbon (DLC) thin film bioelectrodes: Effect of thermal post-treatments and the use of Ti adhesion layer. Tomi Laurila, Antti Rautiainen, Sakari Sintonen, Hua Jiang, Emilia Kaivosoja, Jari Koskinen, 34, 446–454, 2014. doi: 10.1016/j.msec.2013.09.035
- Enzyme Based Amperometric Biosensor for Adenine Determination. Beáta Bóka, Nóra Adányi, Diána Virág, Ivo Frebort, Attila Kiss. Electroanalysis, 25, 237-242, 2013. doi: 10.1002/elan.201200387
- Influence of anode potentials on selection of Geobacter strains in microbial electrolysis cells. Audrey S. Commault, Gavin Lear, Michael A. Packer, Richard J. Weld. Bioresource Technology, 139, 226–234, 2013. doi: 10.1016/j.biortech.2013.04.047
- Simultaneous measurements of glucose, oxyhemoglobin and deoxyhemoglobin in exposed rat cortex. R. Roche, P. Salazar, M. Martín, F. Marcano, J.L. González-Mora. Journal of Neuroscience Methods, 202, 192–198, 2011. doi: 10.1016/j.jneumeth.2011.07.003
- Micro-ring disc ultramicroelectrodes array for direct detection of NO-release from S-nitrosoglutathione. Loan To Thi Kim, Aurélie Girard, Laurent Griscom, Florence Razan, Sophie Griveau, Fethi Bedioui. Electrochemistry Communications 13, 681-684, 2011. doi: 10.1016/j.elecom.2011.04.008
- Improvement and characterization of surfactant-modified Prussian blue screen-printed carbon electrodes for selective H2O2 detection at low applied potentials. P. Salazar, M. Martín, R.D. O’Neill, R. Roche, J.L. González-Mora. Journal of Electroanalytical Chemistry, 674, 48-56, 2012. doi: 10.1016/j.jelechem.2012.04.005
- Spoilage Detection with Biogenic Amine Biosensors, Comparison of Different Enzyme Electrodes. Beáta Bóka, Nóra Adányi Diána Virág, Marek Sebela, Attila Kiss, Electroanalysis, 24, 181-186, 2012. doi: 10.1002/elan.201100419
- Voltammetric Determination of Dissolved Nitrous Oxide. Natalija Veli, Mirela Samardzi, Milan Sak-Bosnar, Bozidar Šantek. International Journal of Electrochemical Science, 6, 1206-1215, 2011. electrochemsci.org/papers/vol6/6041206.pdf
- Enzyme-based choline and l-glutamate biosensor electrodes on silicon microprobe arrays. O. Frey, T. Holtzman, R.M. McNamara, D.E.H. Theobald, P.D. van der Wal, N.F. de Rooij, J.W. Dalley, M. Koudelka-Hep. Biosensors and Bioelectronics, 26, 477–484, 2010. doi: 10.1016/j.bios.2010.07.073
- Development of an implantable d-serine biosensor for in vivo monitoring using mammalian d-amino acid oxidase on a poly (o-phenylenediamine) and Nafion-modified platinum–iridium disk electrode. Zainiharyati M. Zain, Robert D. O’Neill, John P. Lowry, Kenneth W. Pierce, Mark Tricklebank, Aidiahmad Dewa, and Sulaiman Ab Ghan. Biosensors and Bioelectronics, 25, 1454-1459, 2010. doi: 10.1016/j.bios.2009.10.049
- Simultaneous neurochemical stimulation and recording using an assembly of biosensor silicon microprobes and SU-8 microinjectors. Olivier Frey, Tahl Holtzman, Ruth M. McNamara, David E.H. Theobald, Peter D. van der Wal, Nico F. de Rooij, Jeff W. Dalley, and Milena Koudelka-Hep, Sensors and Actuators B, 154, 96-105, 2011. doi: 10.1016/j.snb.2010.01.034
- Real-time electrochemical detection of extracellular nitric oxide in tobacco cells exposed to cryptogein, an elicitor of defence responses. Angelique Besson-Bard, Sophie Griveau, Fethi Bedioui, and David Wendehenne. Journal of Experimental Botany, 59, 3407-3414, 2008. doi: 10.1093/jxb/ern189
- A disposable two-throughput electrochemical immunosensor chip for simultaneous multianalyte determination of tumor markers. Jie Wu, Zhijie Zhang, Zhifeng Fu, and Huangxian Ju. Biosensors and Bioelectronics, 23, 114–120, 2007. doi: 10.1016/j.bios.2007.03.023
Quadstat Potentiostat
Maximum control voltage: ±2.5 V (±10 V with external input)
Maximum current per channel: ±10 mA
Compliance voltage: >10 V
>5 V on 10 mA range (high current model)
Input resistance: 1013 Ω || 1 pF
Input bias current: <1 pA @ 25 °C
Current range settings: ±10, 5, 2, 1 mA
±500, 200, 100, 50, 20, 10, 5, 2, 1 μA
±500, 200, 100, 50, 20, 10, 5, 2, 1 nA
±500, 200 pA
I/V Gain: 106, 105, 104, 103, 100, 10, 1, 0.1 nA/V
DC current error: < ±1% FS on ranges 200 nA – 10 mA
< ±0.5% FS on ranges 200 pA – 100 nA
Current signal offset: ±5000 μA on ranges 2 μA – 10mA
±5 μA* on ranges 20 nA – 1 μA
±50 nA* on ranges 200 pA – 10 nA
Low-pass filter: 10 Hz, 3rd order Bessel
e-corder filter settings: 10 kHz to 1 Hz in 10:5:2 steps
Bandwidth, unfi ltered: >10 kHz, on ranges of 20 nA – 10 mA
>1 kHz, on ranges of 200 pA – 10 nA
Drift with temperature: <10 μV/°C
I2C input and output: Male and female DB-9 pin connectors.
Provides control and power.
More Information
EA164_QuadStat
(220 KB PDF)
Also see:
ES500 Chart and Scope Software
ES260 EChem Electrochemistry Software for Voltammetric Techniques
ET014 EChem Electrode Kit